Characterization and modeling of thermo-mechanical fatigue crack growth in a single crystal superalloy
Turbine engine blades are subjected to extreme conditions characterized by significant and simultaneous excursions in both stress and temperature. These conditions promote thermo-mechanical fatigue (TMF) crack growth which can significantly reduce component design life beyond that which would be predicted from isothermal/constant load amplitude results. A thorough understanding of the thermo-mechanical fatigue crack behavior in single crystal superalloys is crucial to accurately evaluate component life to ensure reliable operations without blade fracture through the use of “retirement for cause” (RFC). This research was conducted on PWA1484, a single crystal superalloy used by Pratt & Whitney for turbine blades.
Initially, an isothermal constant amplitude fatigue crack growth rate database was developed, filling a void that currently exists in published literature. Through additional experimental testing, fractography, and modeling, the effects of temperature interactions, load interactions, oxidation and secondary crystallographic orientation on the fatigue crack growth rate and the underlying mechanisms responsible were determined. As is typical in published literature, an R Ratio of 0.7 displays faster crack growth when compared to R = 0.1. The effect of temperature on crack growth rate becomes more pronounced as the crack driving force increases. In addition secondary orientation and R Ratio effects on crack growth rate were shown to increase with increasing temperature. Temperature interaction testing between 649°C and 982°C showed that for both R = 0.1 and 0.7, retardation is present at larger alternating cycle blocks and acceleration is present at smaller alternating cycle blocks. This transition from acceleration to retardation occurs between 10 and 20 alternating cycles for R = 0.1 and around 20 alternating cycles for R = 0.7. Load interaction testing showed that when the crack driving force is near KIC the overload size greatly influences whether acceleration or retardation will occur at 982°C. Semi-realistic spectrum testing demonstrated the extreme sensitivity that relative loading levels play on fatigue crack growth life while also calling into question the importance of dwell times. A crack trajectory modeling approach using blade primary and secondary orientations was used to determine whether crack propagation will occur on crystallographic planes or normal to the applied load.
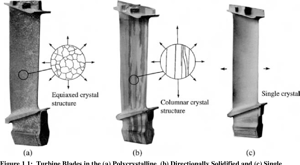
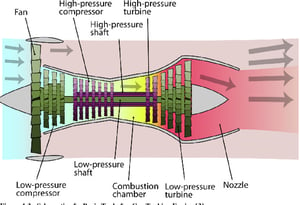
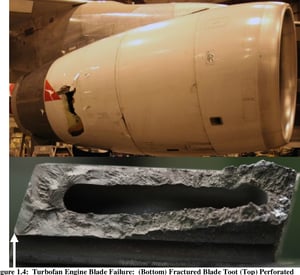
Crack plane determination using a scanning electron microscope enabled verification of the crack trajectory modeling approach. The isothermal constant amplitude fatigue crack growth results fills a much needed void in currently available data. While the temperature and load interaction fatigue crack growth results reveal the acceleration and retardation that is present in cracks growing in single crystal turbine blade materials under TMF conditions. This research also provides a deeper understanding of the failure and deformation mechanisms responsible for crack growth during thermo-mechanical fatigue. The crack path trajectory modeling will help enable “Retirement for Cause” to be used for critical turbine engine components, a drastic improvement over the standard “safe-life” calculations while also reducing the risk of catastrophic failure due to “chunk liberation” as a function of time. Leveraging off this work there exists the possibility of developing a “local approach” to define a crack growth forcing function in single crystal superalloys.
Author: Benjamin Scott Adair, Dissertation; George W. Woodruff School of Mechanical Engineering; Georgia Institute of Technology; August, 2013