Abstract
Objectives
Oxygen tension affects the biology of aerobic and denitrifying organisms. Using a novel, fast‐response sensor, we developed a noninvasive procedure to measure pO2 in distal human airways. We hypothesized that distal pO2 would be low in cystic fibrosis (CF) airways.
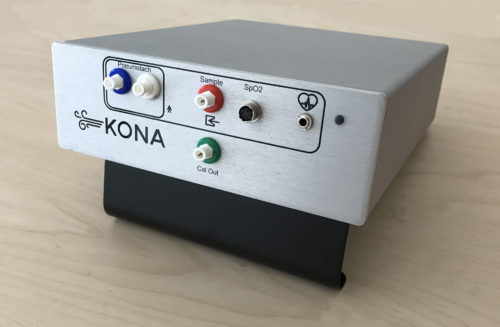
Materials and Methods
We measured the fraction of expired oxygen (FEO2) in real time using a fast laser diode analyzer in healthy subjects and in patients with CF, asthma, and primary ciliary dyskinesia (PCD). Subjects slowly exhaled to residual volume (RV), where the nadir of FEO2 (NFO) was recorded. Values were compared to peripheral oxygen saturation (SaO2), expired CO2 at RV, FEV1, FEV1/FVC, and FEF25‐75. We also measured the effect of supplemental oxygen on FEO2.

Results
Seventy‐four subjects completed the study. Seven additional subjects could not perform the maneuver. Mean (±SD) NFO values for controls (n = 29), CF patients (n = 23), asthma patients (n = 15), and PCD patients (n = 7) were 13.4 ± 1.1%, 12.4 ± 1.2%, 13.3 ± 1.1%, 14.4 ± 0.6%, respectively. NFO in CF was lower than in controls (P = 0.0162), and NFO in PCD was higher than in CF (P = 0.0007). Asthma results were heterogeneous. Oxygen caused a dose‐dependent increase in NFO (P < 0.0005; n = 3; r2 = 0.91). NFO values were positively associated with FEV1 (P = 0.0009), FEV1/FVC (P = 0.0019) and FEF25‐75 (P = 0.0155), but there was no association with SaO2.
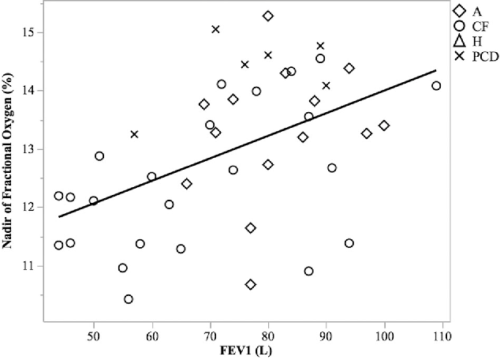
Conclusions
Distal airway pO2 is lower in CF than in controls. This may reflect absorption of oxygen in partially plugged acinar units, and/or increased epithelial oxygen consumption. Distal airway pO2 can be precisely titrated to treat infections.

Authors: Lori Mendelsohn BA(1),(2); Christiaan Wijers BS(1),(2); Ritika Gupta BS(1),(2); Marozkina MD(2); PhD Chun Li PhD(3); Benjamin Gaston MD(1),(2)
(1) Division of Pediatric Pulmonology, RainbowBabies and Children’s Hospital, Cleveland,Ohio
(2) Department of Pediatrics, Division ofPulmonology, Case Western ReserveUniversity, Cleveland, Ohio
(3) Department of Population and QuantitativeHealth Sciences, Case Western ReserveUniversity School of Medicine, Cleveland,Ohio
First published: 28 November 2018; Pediatric Pulmonology
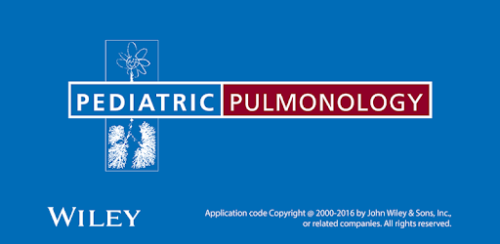
Aircrew hypoxia warning times can be reduced by perfecting laser diode absorption spectroscopy oxygen sensors for OBOGS monitoring.

The onboard oxygen generating system(OBOGS) is an alternative to liquid oxygen (LOX). When compared to a LOX system, the OBOGS has several advantages. First, it’s availability may be as high as 99 percent. There is no requirement for depot-level maintenance. The OBOGS has no daily service requirements, and scheduled preventive maintenance occurs at 2,000 hours. Incorporation of the OBOGS eliminates the need to store and transport LOX. Additionally, it eliminates the need for LOX support equipment. The potential for accidents related to LOX and high-pressure gases is greatly reduced. The basic components of the OBOGS are the concentrator, oxygen monitor, and oxygen breathing regulator. The concentrator produces an oxygen-rich gas by processing engine bleed air through two sieve beds. The oxygen monitor senses the partial pressure of the gas and, if necessary, provides a low-pressure warning to the pilot. The oxygen regulator is a positive pressure regulator.

Pilot Hypoxia:
- Pilot hypoxia can result in impaired judgment, loss of aircraft, and loss of aircrew.
- 22 aircrew hypoxia reports investigated by NAWCAD over 2 years.
- F/A 18C Class A mishap, May 2001, loss of pilot/aircraft, $30M cost of aircraft and site cleanup.

Oxigraf Fast Responding Oxygen Sensors:
- Reliable VCSELs now make laser diode oxygen sensors viable for air crew OBOGS monitors.
- Effort can be combined with OBOGS flow/pressure monitor for integrated pilot “dry mask” warning or backup system.
- LD Sensor fast enough to monitor gas composition blender systems.
Please download your On-Board Oxygen Generating System (OBOGS) Monitoring Application Note (pdf)
The Oxigraf team recently worked with a client to provide them with a reliable, high-quality linear acceleration tunnel oxygen deficiency sensor. A detailed case study is outlined below.
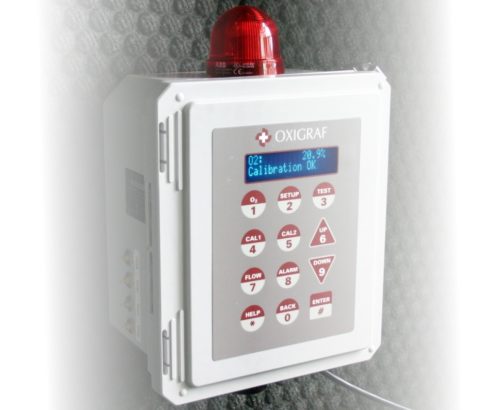
The Problem
A customer was in need of a reliable solution for sampling gas from remote locations in a tunnel in order to monitor equipment and personnel safety. During their operations, they were dealing with radiation hazards, which posed risks for electronics, and during maintenance periods, they were dealing with the presence of nitrogen and helium, which presented oxygen deficiency hazards. Oxygen deficiency in the workplace can lead to blackouts, cause falls, and present more serious health risks — some of which can be fatal. The Oxigraf team was brought in to help them eliminate the risk of oxygen depletion.
The Solution
After assessing the clients’ unique needs, we opted to provide them with our Oxigraf Model O2iM, which has a high-flow pump option and allows for sampling from long distances. This sensor allows for continual monitoring of the clients’ tunnel atmosphere from a safe location, and provides local alarms and interfaces with sophisticated safety features to prevent hazards such as cryogenic spills, which can lead to rapid displacement of breathing air. Oxigraf’s top-of-the-line oxygen deficiency monitor is flexible and efficient, and provided the client with a reliable, immediate oxygen alarm for concentrations of less than 19.5%. It also eliminated the need for frequent recalibration or replacement of oxygen sensors, as well as the comprehensive, time-consuming maintenance often involved in sampling systems. The risk of false alarms and alarm failures was also eliminated.
This unique sensor features a rapid response time of less than a second. The transit time of the gas sample through the sampling tube may be 1 second per meter of sampling tube, and to respond within 5 seconds, an oxygen monitoring alarm with a 1-second response time can be placed within 4 meters of the potential hazard. The built-in pump draws gas remotely, allowing for these quick response times. In fact, we offer the best response speed/signal in the industry, and can add multiplexors (valving) in order to monitor four or more locations from over 100 feet away.
Additionally, this sensor is insensitive to movement, has auto-calibration for absolute accuracy, and includes options for multi-port and high-flow sampling. It also features a remote display and battery backup to allow for proper functioning during power interruptions. In addition, it can be fitted with a Z-Purge system, which allows the unit to be used in Class 1 Div 2 hazardous areas. The monitor includes a sampling pump, hydrophobic filter, and flow sensor, while the microprocessor controller maintains the flow at a constant value.
The Result
The customer compared our O2iM sensor to other O2 sensing solutions and determined that O2iM was “the champion,” allowing for reliable performance 24/7. They were especially impressed with the unique engineering of the “Pre-Fetch” high-flow pump option, which allows for the monitoring of distant sample locations while maintaining fast response times.
Typical O2iM Installation:

Learn More
Oxigraf has over 15 years of experience producing laser gas sensors and instruments, and is the leading manufacturer of laser absorption spectroscopy sensors for oxygen gas measurement and analysis. Oxigraf O2iM Oxygen Safety Monitors have been widely adapted in hundreds of facilities since 2004, replacing a wide range of less reliable electrochemical sensors. Oxigraf O2 and CO2 sensors, in particular, have been widely adapted by OEM manufacturers of medical respiratory gas monitors in order to measure breath waveforms, end-tidal gas values, anaerobic thresholds, VO2 maxs, and non-invasive cardiac outputs. For more information on our sensors, or to speak with an expert about your specific monitoring needs, contact the team today.
Please download your Oxigraf Case Study: Linear Acceleration Tunnel Oxygen Deficiency Sensor